2.1 Types of Energy
This submodule examines energy, specifically how energy is transferred from the Sun to the Earth. A good place to start with this topic is with definitions.
Energy is traditionally described as the ability to do work. The unit of energy is a joule (pronounced “jewel”). One joule is equal to 0.237 calories. This “calories” is the same “calories” found on the nutritional labels of food. Eating food or calories provides energy for a person to do work.
Radiation is the emission and transfer of energy via particles or waves. Many people think of radiation as coming from nuclear reactors or bombs. This type of radiation transfers energy with subatomic particles. In addition, an everyday light bulb, radio, and microwaves are all examples of devices that produce and use radiation. The type of radiation in these examples uses waves, there is no mass or charge but there is energy. The energy packets in the waves are called photons. The entire range of these waves is called the electromagnetic spectrum.
How is the electromagnetic spectrum created? You probably know that the building blocks of all matter are atoms and molecules. Within these atoms and molecules are smaller particles that have positive and negative charges — protons and electrons. These charged particles tend to oscillate or vibrate. Without getting into more detail, physics tells us that any charged particle like an electron has an electrical field surrounding it. Furthermore, moving charges also possess magnetic fields. Thus, when an electron oscillates, its surrounding electric and magnetic fields change. Like moving your hand rapidly back and forth in a pool of water, oscillating electrons send out ripples of energy (that is, “waves”) that have both electrical and magnetic properties Hence, the name electromagnetic radiation.
The last definition needed is thermodynamics. Studying the movement of molecules and their energy for an atmosphere is overwhelming. Instead, thermodynamics is studied. Thermodynamics examines the relationship between heat and energy. Understanding aspects of thermodynamics help in determining why the atmosphere is stable and at other times when it is not.
2.1.1 Looking at Energy and Thermodynamics
Energy has many forms such as electrical, nuclear, or chemical. All forms of energy fall into the two categories of kinetic and potential. Kinetic energy is a result of motion. Some examples of kinetic energy are the Sun (electromagnetic radiation), moving water, electricity, the burning of fuel or the vibration of molecules which is measured as temperature. This video clip illustrates kinetic energy.
Potential energy is stored and has the capacity to do work under the correct conditions. Some examples of potential energy are water behind a dam, gasoline, food, a battery, or firewood. Potential energy is frequently converted into kinetic energy when work is performed. This video clip illustrates potential energy.
Energy, heat and temperature are all related but not identical to each other. Thermodynamics helps define the relationship between them, and has two laws which are important. The First Law of Thermodynamics states that energy is a conserved property that is neither created nor destroyed. Essentially energy is converted from one form to another. This concept is also called the conservation of energy.
Temperature is a measure of the heat of an object. At a molecular level, temperature measures how fast the molecules are moving or vibrating. Air with a high temperature has gas molecules moving much faster than colder air. The Second Law of Thermodynamic states that heat is always transferred from hot to cold objects. Hot objects tend to cool down and cold objects warm up.
Two temperature scales are widely used, Fahrenheit and Celsius. Fahrenheit has the temperature at which water freezes as 32oF, where as this temperature in Celsius is 0oC. The boiling point of water at a standard air pressure is 212oF and 100oC.
Thermodynamics is an important concept when studying the atmosphere because the Sun transfers energy to the Earth. The Earth in turn transfers energy to the atmosphere in the form of heat. How does this transfer of heat occur? The next section examines this question.
2.1.2 Transfer of Heat Energy
The heat source for our planet is the Sun. Energy from the sun is transferred through space and through the earth’s atmosphere to the earth’s surface. Since this energy warms the earth’s surface and atmosphere, some of it is or becomes heat energy. There are three ways heat is transferred into and throughout the atmosphere: conduction, convection, and radiation.
Conduction
Conduction is the transfer of heat energy without the appreciable movement of molecules. The transfer of heat energy occurs by the kinetic energy translating from one particle to another within the materials. Have you ever left a metal spoon in a pot of soup being heated on a stove? After a short time, the handle of the spoon becomes hot. This is due to the conduction of heat energy from molecule to molecule within the spoon. Conduction is a very effective method of heat transfer in metals. However, conduction of heat through air is poor.
Convection
Convection is the transfer of heat by the mixing of a fluid. An example of convection is the heating of water in a pot. As the water heats, water at the bottom becomes hot and rises. As the hot water rises, its space is replaced by cooler water from above. Both liquids and gases are considered fluids. Thus, the atmosphere behaves like a fluid. The mixing of heat in a fluid is associated with buoyancy, which in turn is related to density. Hot air rises because it is less dense than surrounding air. Cold air sinks because it is denser. As the Sun heats the Earth’s surface, the air molecules near the surface gain heat by conduction. This hot air then rises from the surface through convection.
A pot of boiling water illustrates the transfer of heat by convection; the transfer of heat by the mixing of a fluid.
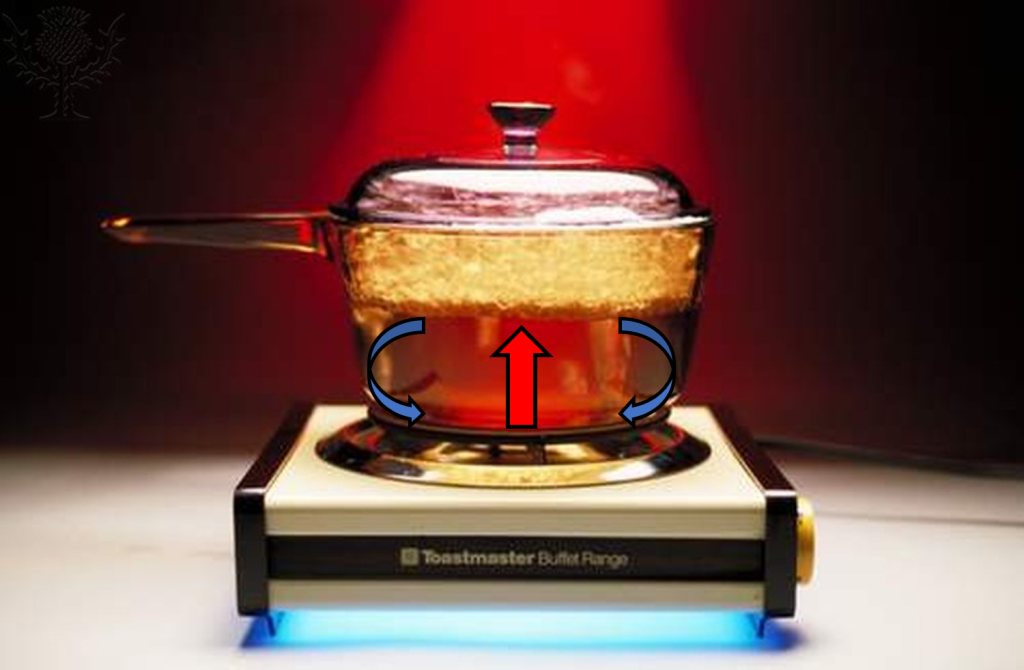
Radiation
The type of radiation most important in studying the atmosphere is electromagnetic radiation. Electromagnetic waves travel from the Sun to the Earth through a vacuum and give energy and life to everything on our planet. From this perspective, the best definition for radiation is the transfer of heat without a transfer medium.
These three methods of heat transfer all occur on our planet.
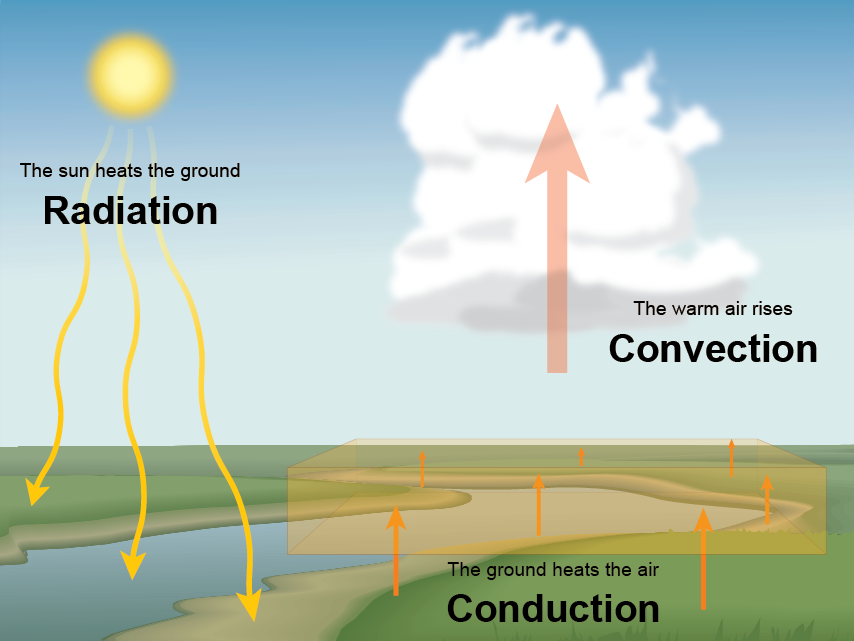
Electromagnetic radiation from the Sun travels to Earth through the atmosphere to heat the ground. As the ground warms, the gas molecules touching the ground warm and start to rise. The rising of hot air mixes this heat throughout the atmosphere.
The details associated with electromagnetic radiation are extremely in warming the Earth and is the primary topic of the next section.
2.1.4 Laws of Radiation
A blackbody is a theoretical object which absorbs all radiation falling upon it. Likewise, blackbodies can also emit a maximum amount of radiation. Both emission and absorption can be applied using the blackbody concept. The emissions from a blackbody are directly related to its temperature. The concept of a blackbody is useful because the Sun and Earth are close to blackbodies, and follow the laws of radiation.
Scientific laws are generally expressed as mathematical equations. The equation reflects the conditions that occur without exception. Assuming an object has blackbody properties simplifies these equations, which in turn makes understanding them easier.
Stefan-Boltzmann Law
The single variable which determines how much energy a blackbody emits is temperature. The Stefan-Boltzmann Law is expressed as
I = σT4
The intensity of radiation (I) equals a constant (σ) times temperature (T) to the fourth power. The constant (σ) is 5.67 x 10-8 watts/m2K4. Temperature is given in degrees Kelvin. Basically, this equation indicates that the higher the temperature the more energy (in watts) an object emits.
Putting some numbers into the Stefan-Boltzmann Law, the average temperature of the Earth is 289oK (16oC and 61oF). Therefore, the Earth emits about 395 watts/m2. The Sun has a much higher average temperature of 5800oK. This temperature gives an emission value of about 64 million watts/m2.
Wein’s Law
Wein’s (pronounced “weens”) Law is another useful law or radiation and states that the wavelength of an object’s peak emission is inversely related to its temperature. The equation expressing this relationship is
λmax = c / T
The wavelength of energy emitted with the greatest intensity is λmax. The other side of the equation has a constant (c = 0.0029 for wavelengths measured in meters) and temperature (T), which is in Kelvin. Wein’s Law physically states that the hotter objects emit shorter wavelengths. Placing numbers into this equation gives the wavelength of peak emission of the Sun at 0.5 µm and the Earth at 10 µm.
These laws of radiation are applied in extracting information from weather satellite imagery. The satellite measures the intensity of radiation emitted from a cloud top. This intensity can be translated to temperature through the Stefan-Boltzmann Law. All infrared satellite imagery, like the one below, uses this law to extract and display detailed temperature information.
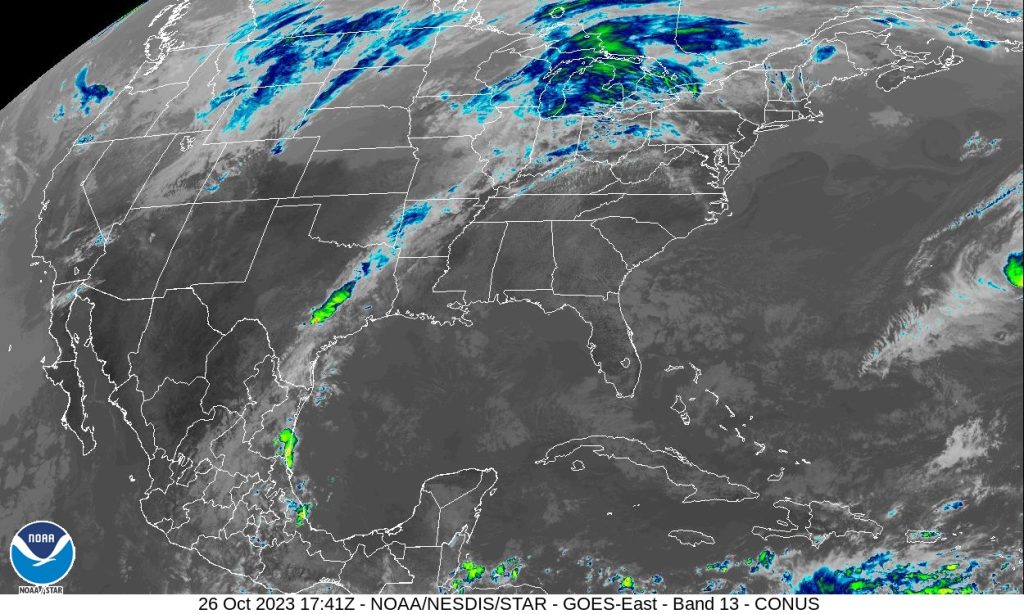
Applying the blackbody concept and these laws of radiation to the Sun-Earth interactions produces this graph. Notice the energy output scale is different for the Sun (left side) and Earth (right side). Both emission curves are assuming blackbody properties. These energy scales are as expected from the Stefan-Boltzmann Law.
The Sun puts out a lot of energy. The shorter wavelengths associated with the Sun, and longer wavelengths from the Earth follow Wein’s Law. A cooler object (the Earth) emits longer wavelengths.
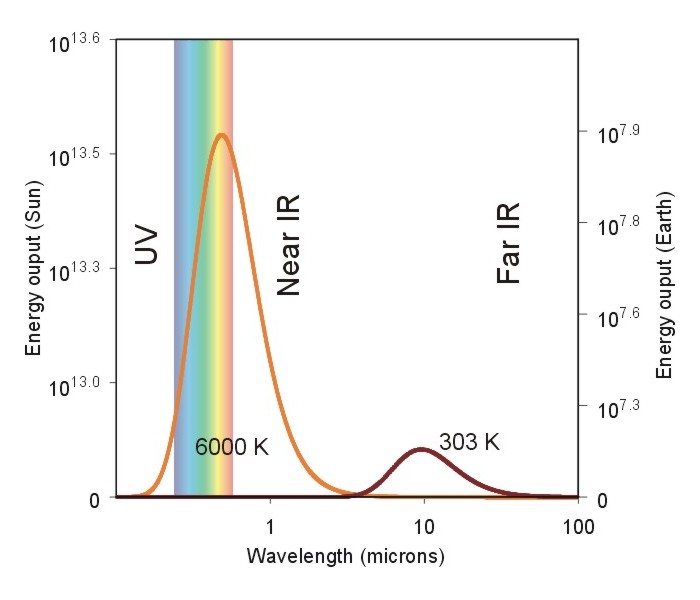
Video: Mod 2.1.4 Laws of Radiation (4:22 min.)
This video discusses blackbodies and the laws of radiation.
2.1.5 How Radiation Interacts with Earth
Previous sections described how electromagnetic radiation behaves and what is emitted by both the Sun and the Earth. A missing piece of information is how the Earth interacts with the radiation received from the Sun. Additionally, we need to know how the atmosphere interacts with incoming radiation from the Sun and outgoing radiation from the Earth. This graph is an expansion of a previous diagram and provides some of these details.
To simplify the terminology describing this physical process, all wavelengths less than 4 µm are referred to as shortwave radiation. Longwave radiation are wavelengths greater than 4 µm. Hence, all incoming radiation from the Sun is shortwave radiation. All outgoing radiation from the Earth is longwave radiation when using this terminology.The gray portion of the bottom graph is what the atmosphere absorbs from the incoming and outgoing radiation. Thankfully, the incoming radiation from the Sun largely passes through the atmosphere. This situation is good in that the energy from the Sun is needed to sustain life on our planet. Notice on the outgoing side, the atmosphere behaves very differently and absorbs much of the energy being emitted by the Earth. The area centered around 10 µm has minimal absorption by the atmosphere and is called a window. Radiation with wavelengths around 10 µm can pass through this window to space and leave the Earth. This absorption aspect of the atmosphere is good! If the Earth were to lose all its heat to space, our planet would be in perpetual winter.What causes this absorption of radiation in the atmosphere? The short answer is the gas molecules composing the atmosphere. The atmosphere is mostly transparent to shortwave radiation from the Sun and opaque to longwave radiation from the Earth. Most of the absorption of outgoing radiation is due to the variable or greenhouse gases (GHGs). Water vapor is the most abundant GHG and and carbon dioxide is a distant second. The other greenhouse gases of ozone and methane also contribute to this absorption. The following chart illustrates the absorption by these atmospheric gases. Notice the absence of absorption by permanent gases. Nitrogen is largely inert in interacting with short and long wave radiation.
The last categories in this chart are nitrous oxide and Rayleigh scattering. Nitrous oxide is a by-product of combustion and is frequently observed as a brownish pollution layer over metropolitan areas. Rayleigh scattering is not a gas, but rather how light interacts with gas molecules. More details on Rayleigh scattering are given later in this module.While the bulk of the Sun’s electromagnetic radiation is in the visible and infrared bands, the Sun can transmit radiation across the entire electromagnetic spectrum. How does the atmosphere interact with wavelengths other than short and longwave radiation? The next diagram answers that question. In this diagram, the purple and red lines extending from the top of the atmosphere to the Earth indicate that the atmosphere is transparent to that band of radiation. If the purple or red lines stop within the atmosphere, absorption is occurring. Gamma, X-ray, and a portion of the ultraviolet spectrum are absorbed in the mesosphere and stratosphere. The far (longer) infrared wavelengths and longer radio waves are also absorbed by the atmosphere. This diagram further illustrates the protective shell the atmosphere provides us since the higher energy portion of the electromagnetic spectrum (purple) is detrimental to life.
Video: Mod 2.1.5 How radiation interacts with the Earth (7:19 min.)
This video discusses how electromagnetic radiation interacts with the atmosphere.
References:
boiling water.png – From ImageQuest: Water boiling. Photography. Britannica ImageQuest, Encyclopædia Britannica, 25 May 2016. quest.eb.com/search/139_1923603/1/139_1923603/cite. Accessed 1 May 2021. Modified to insert convection arrows.
conv_rad_cond.png is from NOAA, NWS and is found at https://www.weather.gov/jetstream/heat
frequency and wavelength.jpg and jump rope analogy.jpg – NASA public domain at https://science.nasa.gov/ems/02_anatomy
EM_sprectrum_compare_level1_lg.jpg – NASA public domain at https://imagine.gsfc.nasa.gov/science/toolbox/emspectrum1.html
emwave.gif and energy_breakdown1.svg – NOAA, NWS public domain at https://www.weather.gov/jetstream/electro
Portions of text from the above websites were used and modified for this page.
NASA at https://solarsystem.nasa.gov/resources/681/solar-system-temperatures/ for Earth’s temperature.
NASA at https://asd.gsfc.nasa.gov/archive/mwmw/mmw_bbody.html for some details on the laws.
shortwave longwave digram.jpg from METEO3 Course Author: Steven Seman (Assistant Teaching Professor, Department of Meteorology and Atmospheric Science, College of Earth and Mineral Sciences, The Pennsylvania State University). Some portions adapted from original course materials by David Babb and Lee M. Grenci. Creative Commons license (cc By-NC-SA 4.0), https://creativecommons.org/licenses/by-nc-sa/4.0/, accessed 3-21-2021 / No modifications.
energy_breakdown2.svg is from NOAA, NWS and is found at https://www.weather.gov/jetstream/absorb
Atmosphere_Transmission.png is from WikiCommons and is found at https://commons.wikimedia.org/wiki/File:Atmospheric_Transmission.png. Looks like its associated with the GNU Free Documentation License.
EM_spectrum_atmosphere.jpg is from NASA and is found at https://imagine.gsfc.nasa.gov/science/toolbox/emspectrum1.html